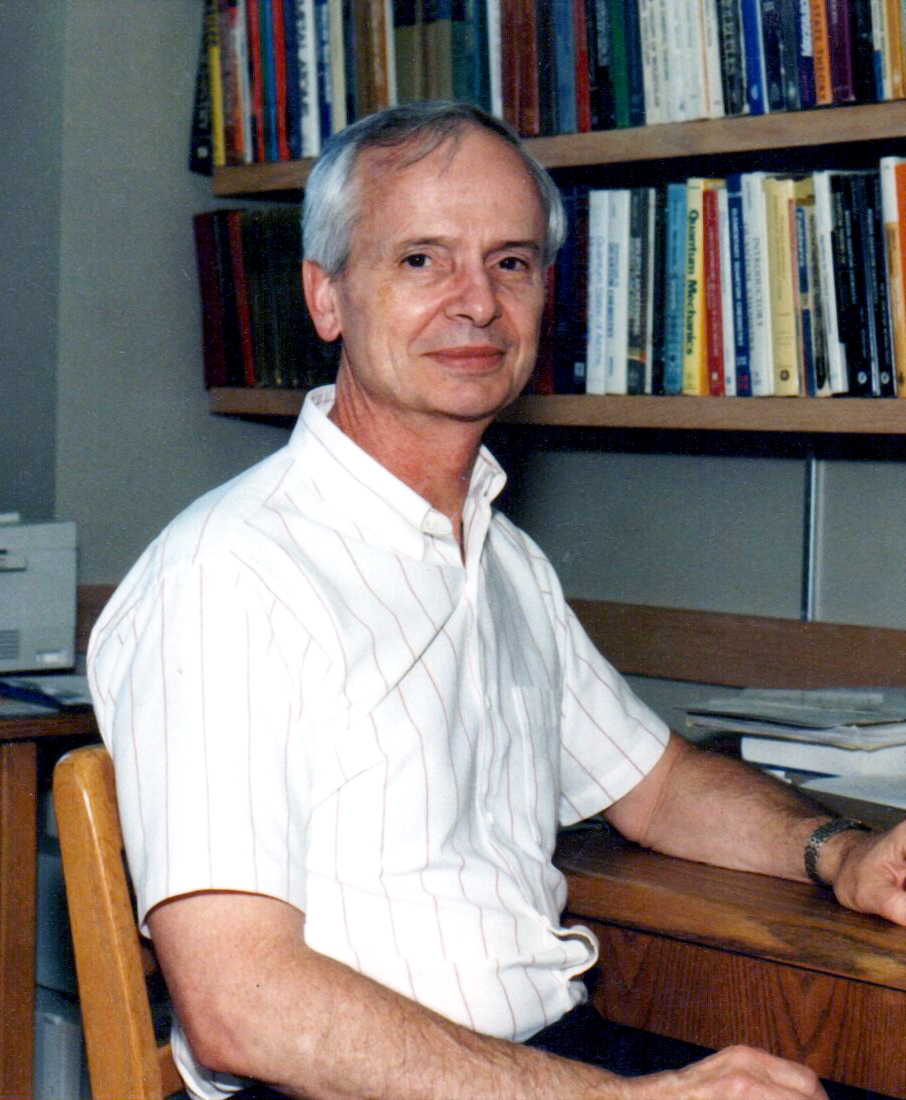
It is now possible to produce accurate wavefunctions for many molecules of chemical interest by using a computer. From this, we can locate the transition states for chemical reactions in the gas phase and determine the reaction paths and activation energies. The information obtained in this way is a useful supplement to what can be found by experiment.
From the wavefunction, we can also determine other molecular properties such as the hyperfine coupling. These properties are useful for interpreting magnetic resonance spectra of radicals and diradicals. Some radicals containing multiple metal centers act as single molecule magnets. We are developing methods for describing these molecules. These new methods are also being used to track chemical reactions such as hydrogen addition to transition metal complexes.
Electronic structures of excited states, reactivity, and spectra can also be computed. The energy levels of excited ions can be computed and compared with the photoelectron spectrum. The momentum distribution of Dyson orbitals can also be computed. This can be compared with the angular distribution of electrons from an (e,2e) experiment.
Calculations on organometallic molecules are still in their infancy. Complete calculations of the equilibrium geometry of transition metal complexes do not always yield satisfactory accuracy, although the electronic structure produced is qualitatively correct. The procedures for predicting transition states and reactions are still in the development stage, and few useful predictions have yet been made.
Many applications of computational quantum chemistry can now be made routinely to predict, interpret, and complement experiment results. But there are still areas where we do not understand the electronic structure of a molecule well enough to allow quantitative prediction of properties. In these areas, computational experiments and new theoretical insights are needed to develop more adequate models.